Solar inverters
Solar inverters, also known as solar power inverters or PV inverters, are specialized electronic devices that convert the direct current (DC) electricity generated by solar panels (photovoltaic or PV panels) into usable alternating current (AC) electricity.
What are solar inverters?
Solar inverters, also known as solar power inverters or PV inverters, are specialized electronic devices that convert the direct current (DC) electricity generated by solar panels (photovoltaic or PV panels) into usable alternating current (AC) electricity. Solar panels generate DC power when exposed to sunlight, and since most of our electrical devices and appliances operate on AC power, solar inverters play a crucial role in enabling the integration of solar energy into the electrical grid and powering homes, businesses, and other facilities.
Here’s how solar inverters work and their key features:
- DC-to-AC Conversion: Solar panels generate DC electricity due to the photovoltaic effect. However, the electricity we use in our homes and businesses is typically AC electricity. Solar inverters convert the DC power generated by solar panels into AC power that matches the grid’s voltage and frequency.
- Maximum Power Point Tracking (MPPT): Many modern solar inverters incorporate MPPT technology. MPPT continuously adjusts the inverter’s operating characteristics to ensure that the solar panels produce their maximum power output under varying conditions like sunlight intensity and temperature. This optimization maximizes the energy harvest from the solar panels.
- Grid Integration: Grid-tied solar systems use solar inverters to synchronize the generated AC power with the utility grid’s power. This allows excess energy to be fed back into the grid, often earning credits or compensation through net metering programs. In times of low solar production, the grid can supply power to the facility as needed.
- Inverter Types: There are different types of solar inverters, each with specific features and applications:
- String Inverters: Commonly used, these inverters handle the output of multiple solar panels wired together in strings.
- Microinverters: These small inverters are installed directly on each solar panel, allowing each panel to operate independently. They are especially useful when panels are shaded or have different orientations.
- Power Optimizers: Similar to microinverters, power optimizers are placed at the back of each solar panel and optimize panel performance.
- Monitoring and Communication: Many solar inverters come equipped with monitoring and communication features. These features allow homeowners or system operators to track the performance of their solar panels and the overall system’s energy production. Some inverters can even provide real-time data and alerts through web interfaces or smartphone apps.
- Safety and Compliance: Solar inverters often include safety features to protect against overvoltage, overcurrent, and other electrical anomalies. They also need to meet specific safety and technical standards set by regulatory authorities.
- System Design: The capacity and type of solar inverter you choose depend on the size of your solar array, the desired level of system monitoring, and the specific requirements of your location and electrical grid.
In summary, solar inverters are essential components in solar power systems. They convert the DC electricity generated by solar panels into AC power suitable for household or commercial use, and they enable grid integration and net metering, helping to make solar energy a viable and efficient renewable energy solution.
DC or Direct current
Direct current (DC) is a type of electrical current characterized by the continuous flow of electric charge in a single direction. Unlike alternating current (AC), where the flow of charge periodically changes direction, DC maintains a constant polarity and remains steady over time. Here are some key features and characteristics of direct current:
Constant Polarity: In a DC circuit, the flow of electrons is unidirectional. Electrons move from the negative terminal (cathode) to the positive terminal (anode) of the power source.
Voltage and Current Relationship: In a DC circuit, the voltage (potential difference) remains constant over time. When a DC voltage is applied to a circuit, a steady current flows through it, governed by Ohm’s law (I = V / R), where I is the current, V is the voltage, and R is the resistance.
Battery and Cell Operation: Batteries and cells are common sources of DC power. They store chemical energy and convert it into electrical energy, producing a continuous flow of electrons in a single direction.
Applications of DC: DC is used in various applications, including:
Batteries and portable electronic devices (smartphones, laptops, etc.).
Automotive systems, including starting and charging systems in vehicles.
DC motors and generators.
Some electronic components and circuits, such as diodes and transistors.
Transmission and Losses: DC has lower losses when transmitted over long distances compared to AC, especially in certain high-voltage transmission scenarios. However, AC is still widely used for long-distance power transmission due to its ability to be transformed and its historical establishment in power grids.
Historical Importance: In the early days of electricity, DC was the predominant form of electrical power distribution. However, the development of AC systems, championed by figures like Nikola Tesla, eventually led to the widespread adoption of AC power distribution due to its efficiency in transforming voltage and its ability to travel long distances.
Conversion to AC: Devices called inverters are used to convert DC power into AC power. In applications like solar power systems and some electronic devices, DC power may need to be converted into AC to match the requirements of the connected loads or the electrical grid.
Polarity and Grounding: DC systems have a defined polarity, meaning there is a clear positive and negative terminal. Polarity is crucial for proper connections and circuit operation. Grounding in DC systems also follows specific conventions to ensure safety and proper functioning.
In summary, direct current (DC) is a continuous flow of electric charge in one direction. It’s characterized by a constant voltage, a specific polarity, and is used in various applications, including batteries, electronics, and certain types of motors. While not as prevalent as alternating current (AC) in modern power distribution systems, DC remains important in many technological areas.
AC or Alternating current
AC, or alternating current on the other hand, is the flow of electric charge such that it constantly reverses direction. The usual form of an AC power is a sine wave. So why do we need AC power? After a brief ‘war of currents’ in the 19th century, AC power was chosen as the standard for central power generation, transmission and distribution. Until this day, almost all national electric grids are AC based. Most households have what is known as the “AC mains socket”. Consequently, most household electric appliances expect to be fed AC power, even though sometimes the internal circuitry of the appliances might use DC. Nevertheless, the fact remains that solar power produced needs to be converted to an AC form so that it is more “usable” in the electricity framework we have today.
Alternating current (AC) is a type of electrical current characterized by its periodic change in direction. Unlike direct current (DC), where the flow of electric charge is unidirectional, AC reverses its direction periodically, creating a sinusoidal waveform. AC is the most common form of electricity used in power generation, transmission, and distribution systems around the world. Here are some key features and characteristics of alternating current:
- Periodic Change in Direction: In an AC circuit, the flow of electric charge alternates between two directions, often referred to as the positive and negative cycles. This reversal of direction occurs at a specific frequency, usually measured in hertz (Hz).
- Sinusoidal Waveform: The voltage and current in an ideal AC system follow a sinusoidal waveform, where the magnitude varies sinusoidally over time. The peak value of the waveform is referred to as the amplitude, and the time taken to complete one full cycle is the period.
- Frequency and Period: The frequency of an AC waveform is the number of complete cycles that occur in one second. It is inversely proportional to the period. In most countries, the standard frequency of the AC power grid is 50 or 60 Hz, depending on regional standards.
- Power Generation and Transmission: AC is the preferred form of electricity for power generation and transmission. Generators at power plants produce AC voltage, which is then transmitted at high voltages over long distances using transformers to minimize energy losses.
- Voltage Transformation: AC voltage can be easily transformed using transformers. This property allows for efficient voltage transmission, as voltage levels can be adjusted to match the requirements of various stages in the power distribution process.
- Capacitors and Inductors: AC has unique interactions with capacitors and inductors. Capacitors store and release energy in response to changing voltage, while inductors store and release energy in response to changing current. These components have important roles in AC circuits.
- Electrical Grids: AC power grids supply electricity to homes, businesses, and industries. The ability to transform and transmit AC voltages efficiently makes it practical to deliver power across vast distances.
- Devices and Appliances: Most electrical devices and appliances designed for household and industrial use operate on AC power. AC motors are particularly well-suited for a wide range of applications due to the alternating nature of the current.
- Conversion to DC: In applications where devices require DC power, such as electronic devices and certain motors, AC power is often converted to DC using devices called rectifiers or power supplies.
- Historical Significance: The development of AC power systems was driven by pioneers like Nikola Tesla and George Westinghouse. AC’s ability to transform voltage and transmit power efficiently played a pivotal role in the “War of the Currents” during the late 19th century.
In summary, alternating current (AC) is a type of electrical current characterized by its periodic reversal of direction. AC power is fundamental to modern power generation, transmission, and distribution systems, and it is the primary form of electricity used for most applications around the world.
How to convert DC to AC for usable electricity?
By using a solar inverter! The inverter converts a DC electrical signal into an AC one. Let’s talk about the features of the solar inverter and its applications in the modern PV system. Solar inverters are classified based on their mode of operation, their size, or implementation topology.
The conversion from DC solar power generated by solar panels to easily usable AC power involves the use of a device called a solar inverter. Solar inverters are designed to perform this conversion efficiently, enabling the integration of solar energy into electrical systems. Here’s how the conversion process works:
- Solar Panels Generate DC Power:
- Solar panels, also known as photovoltaic (PV) panels, absorb sunlight and convert it into direct current (DC) electricity through the photovoltaic effect. The DC power generated by the solar panels is in the form of a constant flow of electrons.
- DC-to-AC Conversion by Solar Inverter:
- The DC power generated by solar panels is not directly usable for most household appliances and devices, which typically operate on alternating current (AC). A solar inverter is used to convert the DC power into AC power that matches the voltage and frequency of the local electrical grid.
- Maximum Power Point Tracking (MPPT):
- Many modern solar inverters incorporate Maximum Power Point Tracking (MPPT) technology. MPPT continuously adjusts the operating characteristics of the inverter to ensure that the solar panels produce their maximum power output under varying conditions, such as changes in sunlight intensity and temperature.
- Conversion Process:
- The solar inverter’s electronics use high-frequency switching to transform the DC power into AC power. The inverter’s control circuitry manages this process to optimize efficiency and ensure that the AC output matches the grid’s voltage and frequency.
- Synchronization with the Grid:
- Grid-tied solar systems use solar inverters to synchronize the generated AC power with the utility grid’s AC power. The inverter ensures that the generated solar power is in phase with the grid power, allowing seamless integration and interaction with the grid.
- Protection and Monitoring:
- Solar inverters often include protection mechanisms to safeguard against overvoltage, overcurrent, and other electrical anomalies. Many inverters also feature monitoring and communication capabilities, allowing homeowners or system operators to track the system’s performance and energy production.
- Output to Electrical System:
- The AC power produced by the solar inverter is then connected to the electrical system of the building or facility. It can be used to power lights, appliances, electronics, and any other devices that require AC power.
- Net Metering (if applicable):
- In grid-tied systems, any excess AC power generated by the solar panels that is not immediately consumed can be fed back into the utility grid. This process often involves net metering, where the utility company credits the system owner for the excess energy produced.
By using a solar inverter to convert DC solar power into AC power, solar energy becomes compatible with the existing electrical infrastructure and can be used to power various devices and systems. The choice of solar inverter depends on factors like system size, desired features, and compatibility with the specific solar panel technology being used.



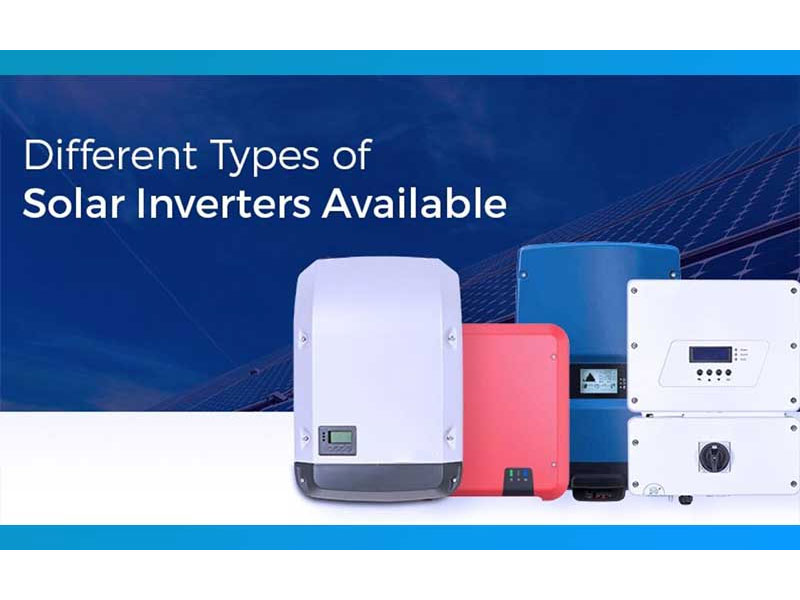
Solar inverter’s classification
There are inverters for grid-connected systems, ones for stand-alone systems and inverters that can be applied in situations that require both connection types, called bimodal or hybrid inverters. The first two types are the most common ones.

Solar grid-tie inverter systems
A grid-tied solar system, also known as an on-grid solar system, is a type of solar power setup that is connected to the local electrical grid. When your solar system generates excess electricity, it’s often credited to your account through a process called net metering. This means that during times when your solar panels produce more electricity than you’re consuming, you effectively “sell” the excess power back to the grid. Overall, a grid-tied solar system allows you to harness solar energy to reduce your reliance on traditional sources of electricity, save on energy bills, and contribute to a more sustainable energy future. One limitation of a grid-tied system is that it won’t provide power during grid outages. This is because grid-tied systems are designed to automatically shut down when the grid goes down to prevent sending electricity back to a potentially damaged grid, ensuring the safety of utility workers. Grid-tied systems are relatively straightforward to install and maintain, as they don’t require the complex battery storage setups that off-grid systems do. With a grid-tied system, you still receive electricity from the grid when your solar panels aren’t producing enough power to meet your needs, such as at night or on cloudy days. You’ll typically still receive a monthly electricity bill, but it may be significantly reduced due to the energy your solar panels contribute. The solar panels generate direct current (DC) electricity from sunlight. An inverter then converts this DC electricity into alternating current (AC), which is the type of electricity used in most homes and businesses.
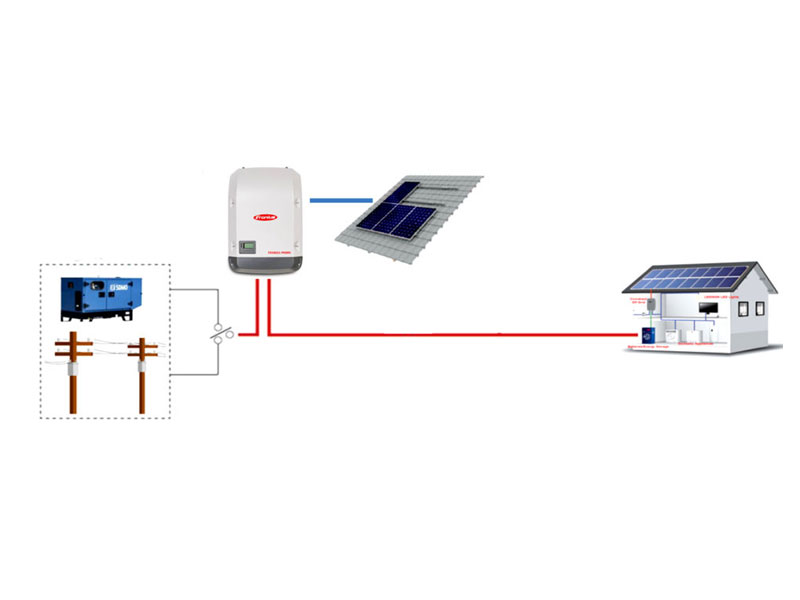


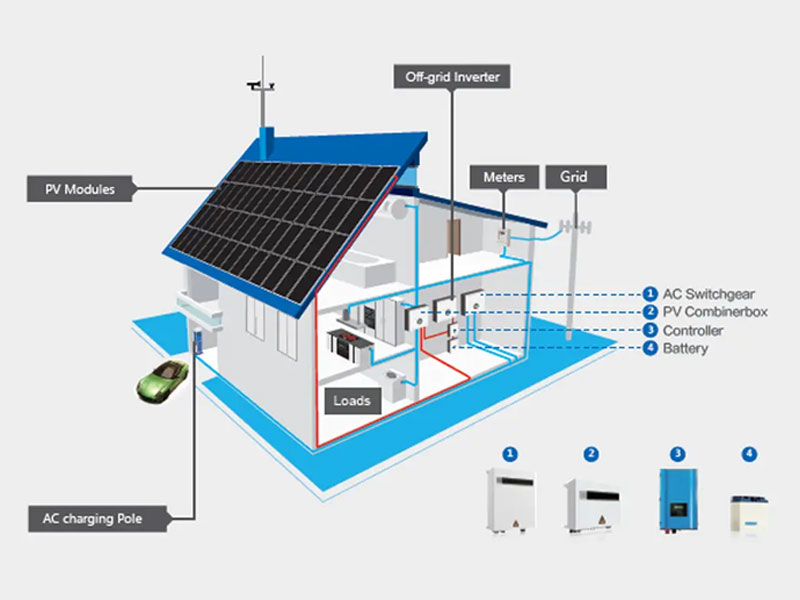
Solar off-grid inverter systems
An off-grid solar inverter system is a self-contained solar power setup that operates independently of the electrical grid. It’s designed to provide electricity to homes, cabins, remote locations, or anywhere where grid power is unavailable or unreliable. A charge controller regulates the flow of electricity from the solar panels to the batteries. It prevents overcharging and optimizes battery life. Energy generated by the solar panels is stored in batteries for use when the sun isn’t shining, such as at night or on cloudy days. Unlike grid-tied inverters, an off-grid inverter converts the DC electricity from the batteries into usable alternating current (AC) electricity that can power household appliances, lights, and other devices. The AC electricity produced by the off-grid inverter powers your home’s electrical needs. This includes appliances, lights, electronics, and other devices. Managing energy usage is crucial in off-grid systems. Users need to be mindful of their energy consumption to ensure they don’t deplete the battery storage too quickly. Off-grid systems require careful planning and sizing to ensure they can meet energy demands throughout the year. Overestimating or underestimating energy needs can lead to inefficient use of resources. Overall, off-grid solar inverter systems provide a reliable and sustainable way to generate and use electricity in locations where grid power is not available or practical. They offer energy autonomy and the opportunity to embrace a more self-sufficient and eco-friendly lifestyle.


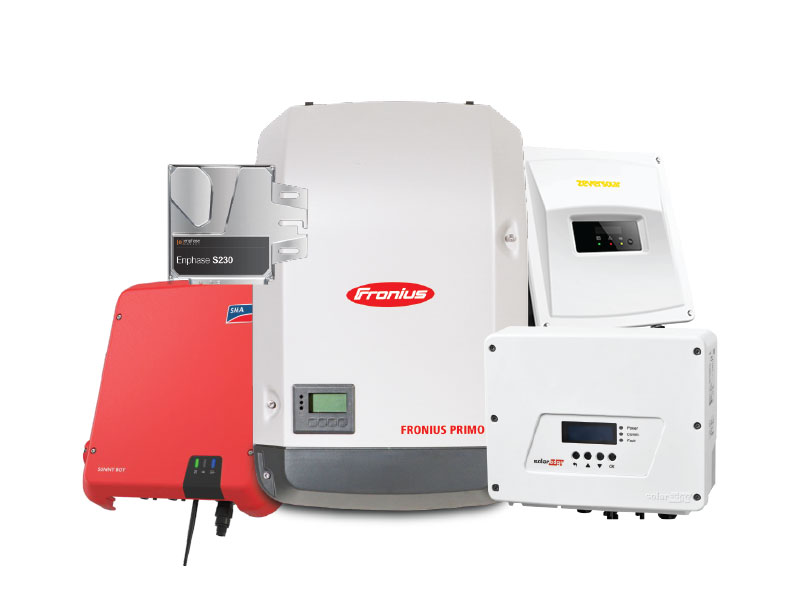
Can the solar inverter perform any additional function?
The answer is yes! Thanks to the advances in power electronics, it is common to have solar inverters that implement a maximum power point tracking or MPPT mechanism before inverting the voltage, thus ensuring that the PV modules or arrays are operating at their maximum power point or MPP. Apart from the modes of operation, solar inverters are also classified on the basis of the implementation topology. There can be 4 different categories under this classification: Central Inverters, which are usually around several kW to 100 MW range. Module inverters, or microinverters, typically rated around 50 to 500W. String inverters, typically rated around 500W to a few kW. A string is nothing but a number of PV modules connected in series. And finally, Multi string inverters, typically rated around 1kW to 10kW range.


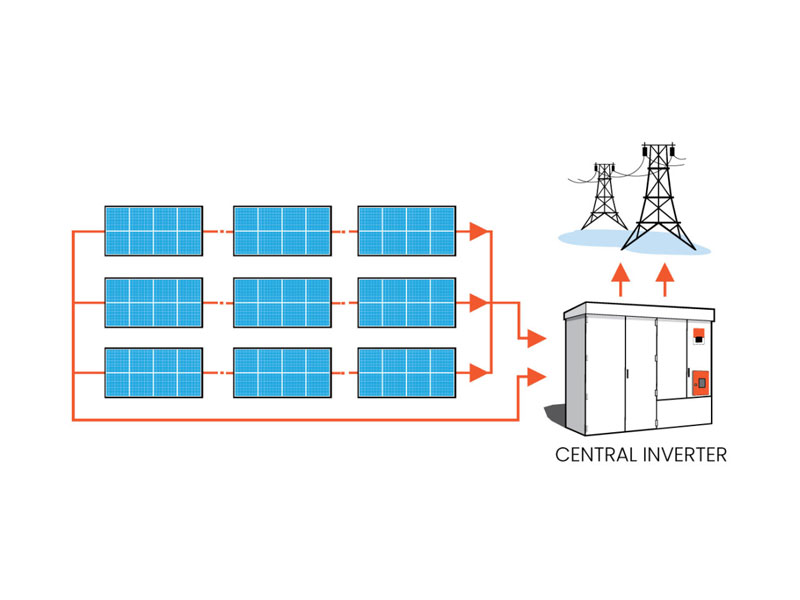
Solar central inverter
This is the most traditional solar inverter topology in use. This is a simple implementation with one solar central inverter catering to all the PV modules in a PV system. While this inverter topology increases the ease of system design and implementation, it suffers from several drawbacks. In large systems, large amounts of DC power will be transferred over long cables to reach the solar central inverter. This increases DC wiring costs, and also decreases safety, as DC fault currents are difficult to interrupt. An MPPT implementation inside the central inverter will only cater to the entire system as a whole. If the various modules, strings are mismatched, let’s say due to partial shading, the overall system output is drastically reduced. Also, the system is usually designed for a fixed power. There is little scope for the extensibility of the system if more strings and modules need to be added.


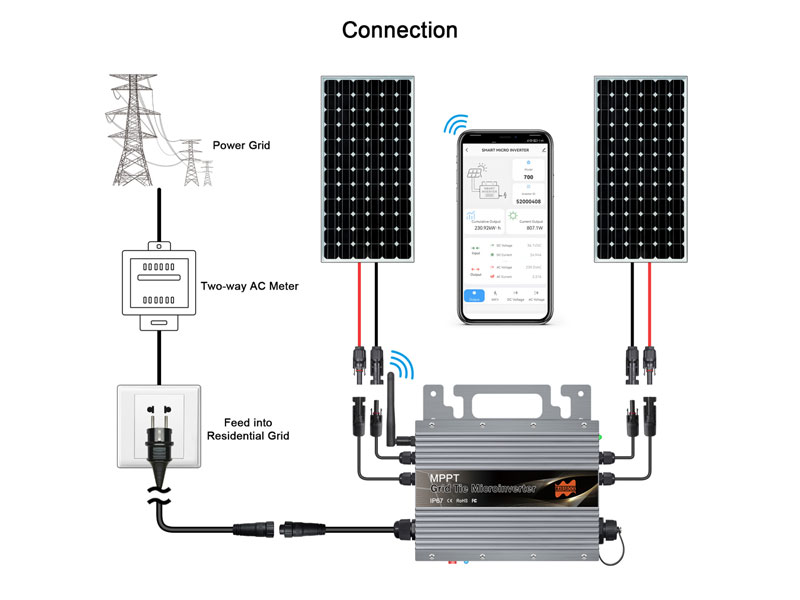
Micro solar inverters
As the name suggests, each module has a dedicated solar inverter with an MPP tracker. Therefore, the topology is more resilient to partial shading effects as compared to the central inverter topology. Clearly, the micro solar inverters provide the highest system flexibility, since extending the size of a system under this topology is far simpler. Furthermore, the DC wiring costs are greatly reduced. However, the investment and maintenance costs tend to increase, especially if the cost per Watt are compared.


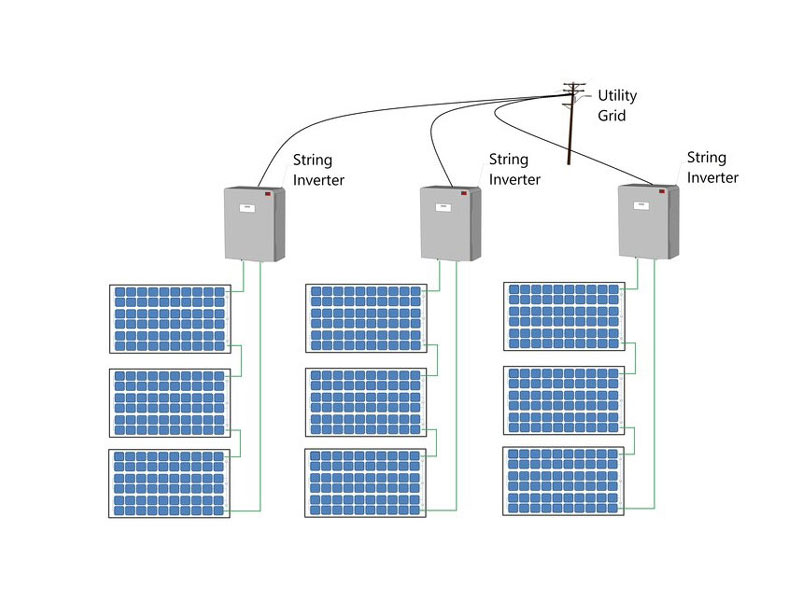
String solar inverters
Then we have the string inverter concept, which seeks to strike a balance between the module level inverter and the central inverter topologies. The string inverter topology is more resilient to mismatch than the central inverter, because each string is independently operated at its MPP, thus guaranteeing a higher energy yield. String inverters are smaller than central inverters. However, the implementation is more complex than the module inverter. Also, the partial shading will have a greater influence over the string inverter topology than over the micro inverter topology.


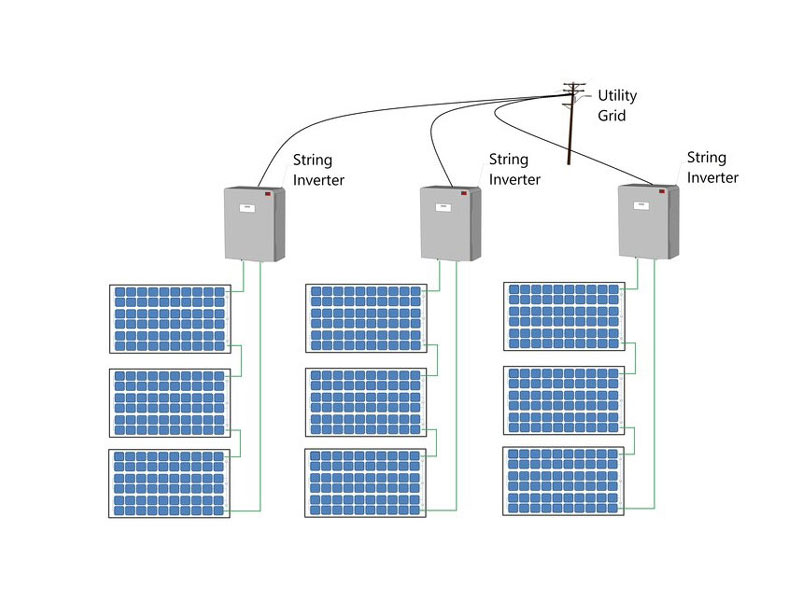
Multi String inverters
This concept seeks to combine the higher energy yield of a solar string inverter with the lower costs of the central inverter. Each of the strings are pre-power-processed using low power DC-DC converters. Each string has its own MPP tracker implemented alongside the DC-DC converter. All the converters are connected via a DC bus to the solar inverter, and ultimately to the grid. Within a certain power range, only a new string with a dedicated DC-DC converter has to be included to expand the system size.


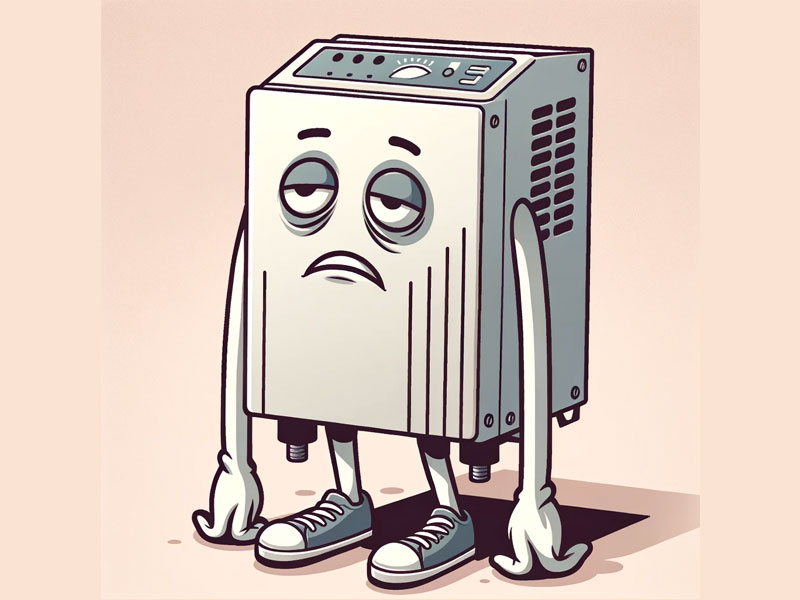
Solar inverter lifespan
It is a work in progress to increase the lifespan of the solar inverter, the crucial power electronic device in the modern PV system. A good solar inverter will probably reach, under favourable conditions, around 10-12 years of lifetime. This is a bottleneck in the modern PV system’s lifetime, especially considering the fact that PV modules can last over 25 years. So now you know what an inverter is, what it does, and how it can be applied in the different system topologies.